Running in parallel to the time-bearing plot, another team of plotters used the same sonar bearings at discrete intervals to geographically trace the progress of bearing drift. The bearing drift appeared as spokes in a wheel. Lieutenant Joseph Ekelund and his colleagues saw that with an accurate target speed ruled as distance on a plastic strip, the plotting party could align the strip with bearings in such a manner as to match the ruled marker with bearing progression. This was done by sliding the strip up and down the most recent bearing, assuming a 90-degree angle-on-the-bow, then tilting the strip at various ranges to see if the ruled speed marks would align with previous bearings. In so doing, target angle-on-the-bow would be shown, target course could be derived and range estimated. It was not an exact science, but successive attempts resulted in surprisingly accurate information, particularly when in the hands of plotters who were willing to trust the method. As the plot continued, the initial estimate could be verified to eliminate other possible matches. The Ekelund “speed strips” method was simple in the extreme, but surprisingly effective at a time when electronic computers were far in the future.
Normally, the optimum firing point for the bearings’ only problem was about the same as for the normal periscope attack, with the exception that a greater stress was placed on obtaining near-zero gyros, optimal track angle and a short torpedo run to reduce the effect of not having a perfect solution. Optimum track angles produced a minimized effective target length. The shortest possible torpedo run reduced the affect of inherent errors.
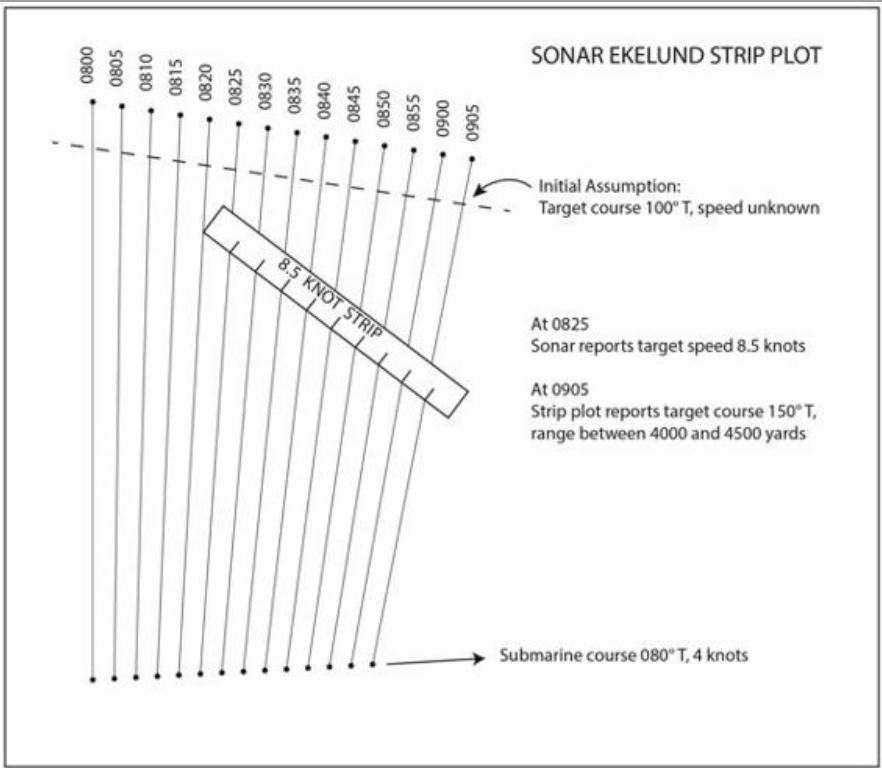
The Ekelund plot depended on accurate sonar bearings taken at consistent intervals. Sonar accuracy was seldom as vivid as portrayed above (Submarine Research Center).
Whether the plots were run in the wardroom, pump room or engine room, the accuracy of problem analysis depended on the acuity of sonar bearings and the ability of sonar operators to identify the unknown submerged vessel as a Soviet submarine, and if so, the type of submarine with corresponding screw noise signature. If bearings could be pinpointed and if the screw noise signature could be correctly identified, the sonar operator had a good chance of obtaining an accurate turn count on the screw noise. He then could enter the turn count into his ship/submarine turn count conversion tables and render a probable target speed.
Target speed was reported to the sonar supervisor, who integrated the information with any other data at hand and told the plot coordinator the best target speed. At precise time intervals target bearings were reported and plotted. An Ekelund plot might appear as follows:
In this example, the listening submarine is on course 080 and the 8.5 knot speed strip reflects sonar’s best estimate of target speed. The grid of the strip aligns with the timed bearings to indicate a target course of 150, range about 4200 yards. Were this an actual approach and the target positively identified as hostile, the captain would either attempt to receive from ComSubLant or ComSubPac approval to begin a tracking mode or would have standing orders relevant to the situation. The captain, acting as approach officer, would be thinking of his TDC set-up (and later the Mark 101 set-up) in relation to the possible use of a Mark 37 torpedo.
During the Second World War the Mark 28 electric torpedo was an alternative to the straight-running, steam-propelled Mark 14 torpedo. Also during the war a purely defensive weapon, the Mark 27, had several unique features. It was the submarine’s first swim-out torpedo. With longitudinal guide rails and a small diameter, the torpedo was pushed silently out of the tube by its propeller. The torpedo then followed a pre-set course to a point where its passive sonar was activated. It was an excellent evasion maneuver weapon. The Mark 37 torpedo design was largely based on the concept of the Mark 27.
In the bearings-only attack, the Mark 37, Mod 4 torpedo was the weapon of choice. The optimum firing point would be reached when the target was within the Mark 37 torpedo’s enabling range of between 600 and 3,100 yards. The standard firing point thumb rule was 2500 yards. Target angle-on-the-bow at time of firing should not have been more than 60 degrees. The Mark 37 torpedo was a relatively slow electric torpedo and a large track angle indicated a probable divergent target course, with accompanying probability of the target outrunning the Mark 37’s capacity to close the target. An angle-on-the-bow of less than 60° at time of firing and a range of 2500 yards or less would maximize the chances of the torpedo’s acoustic acquisition. With a target submarine at a range of over 4000 yards, but with plot showing range decreasing and bearings reasonably constant, the captain would simply stay on course until the target was within range.
Once the torpedo had been launched, the submarine had to take evasive action on the assumption that own ship might be in the cross hairs of the target submarine. The captain knew the condition of his battery, which dictated how much speed he could use in an evasive maneuver. He balanced speed with cavitation noise emission at his depth and normally descended to find a positive gradient. Soviet ASW tactical doctrine of the 1950s called for use of active sonar much more abundantly than did American doctrine. If a Soviet submarine or surface vessel used active sonar in an attempt to locate the attacking submarine, the captain’s best chance of hiding was to get under a layer. The following diagram illustrates the evasion maneuver.
The effectiveness of active sonar to detect an evading submarine is often thwarted by some form of a positive gradient found in about 75 percent of ocean strata. The best depth for a submarine attempting to avoid detection by active sonar above a layer was to descend as quickly as possible. The avoidance of passive sonar was to maintain a slow speed to avoid cavitation. Avoidance of active sonar was to continually present a bow-on or stern-on aspect to the estimated bearing of the hostile submarine and to descend to a depth well below the positive gradient shown on the BT.
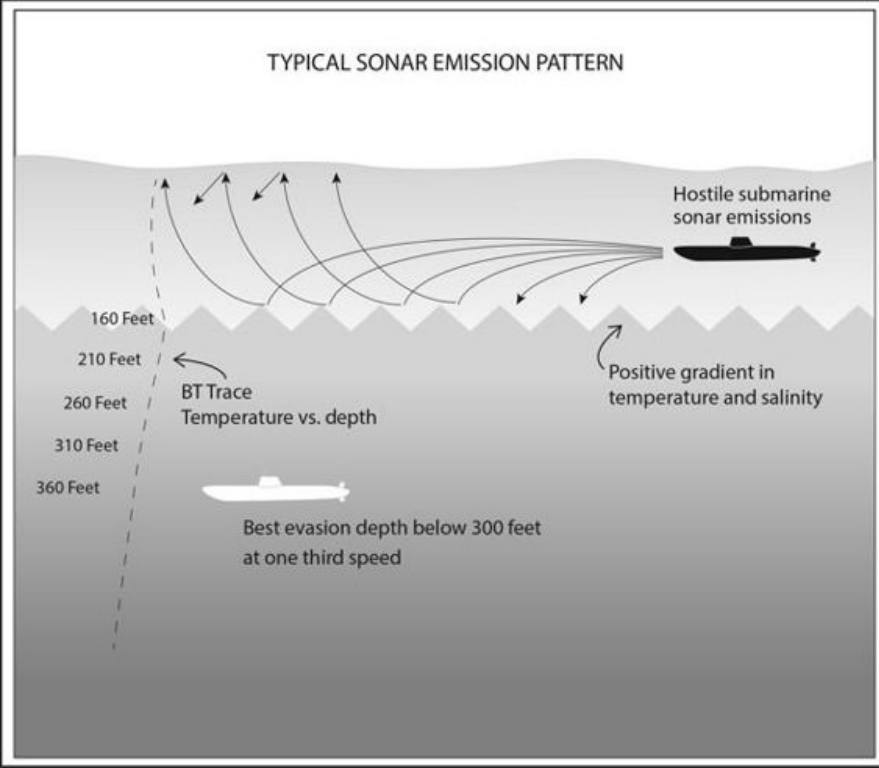
If the hostile submarine dove below the layer depth, the evading submarine could ascend above it and thereby remain hidden from active sonar (Submarine Research Center).
The evading submarine had a variety of maneuvers and tools at hand. The simplest was to release a mass of bubbles while turning the submarine and changing depth. This was the knuckle. At least one innovative boat found that by putting an electric razor to the UQC microphone, the sound in water was strikingly similar to an electric torpedo. This technique was intended to intimidate a hostile boat, but it never saw action against a Soviet submarine. The evasion arsenal included such tools as false-target canisters which could be ejected from the pyrotechnic ejector in the after torpedo room. These were used as the source of bubbles when performing a knuckle. The evasion weapon of last resort was the Mark 27 torpedo, normally carried in the after tubes. This torpedo was only 7 feet, six inches in length and 19 inches in diameter. As described above, it was swim-out launched by having a smaller diameter with guide rails that matched the 21-inch tube diameter. Its run was gyro controlled on a specified course, at the end of which its rudder assumed control, turning the torpedo in a wide circle. When its passive sonar detected a sound source it attacked that source.
While these measures could be deployed, the evading submarine’s best initial tactic when sensing that a hostile submarine had detected its presence was to go to ultra-quiet. Each compartment had a silent running bill and an ultra quiet running bill. In the latter, all machinery and pumps were shut down with the bow and stern planes being shifted to hand power. When such a condition lasted for several hours the planesmen became so exhausted that they would be relieved by off-watch planesmen.
During the 1950s the Navy renewed its effort to silence its submarines. Sound isolation of equipment became the focus of each yard overhaul. Running the diesel engines while snorkeling put so much sound in the water that shore-based low-frequency installations could monitor snorkeling boats as they transited the Atlantic. Neoprene engine mounts were inserted and experiments were conducted to suspend engines within soundproof boxes. Each line carrying fuel, water, hydraulic fluid and electrical conduiting had flexible inserts to reduce machinery vibrations entering the hull. While improvements were made in sound emission control, the GUPPY boats could not overcome the cavitation of the traditional four-bladed screw. Crews relied upon simple solutions: shut down every piece of non-vital equipment, reduce speed and go deep. During a submerged encounter with a Soviet submarine, crew members talked in whispers.
In 1946, the Bureau of Ordnance contracted with Arma Corporation for the design and development of an integrated torpedo fire control system which would augment the capacity of the torpedo data computer Mark IV.
It is difficult, in an age when a computer can be held in the palm of one hand, to visualize the difficulties of engineering a computer when electronics consisted of vacuum tubes and wiring. The evolution of the modern computer, when it is reversed, starts with micro chips the size of a pinhead, chips the size of a postage stamp, circuit boards with transistors and finally vacuum tube-driven monsters the size of a living room. In 1946 computers were made of syncro-servo units, tiny motors driving gears and mechanical displays that required considerable interpretation.
The new system was to incorporate concepts which evolved out of long wartime use of the TDC. Ideas presented to Arma Corporation by experienced submarine officers included an analyzer that could produce a quick, initial target solution, reduction of hand-inserted data with accompanying automation of target data assimilation, and automatic shift of torpedo ballistic data for mixed torpedo loads. Torpedo officers saw the need for electrical transmission of tactical data to tube-loaded torpedoes. The spindle-set torpedo required a mechanical connection with the torpedo tube so that point-of-fire information could be relayed to the torpedo. Experienced submarine officers knew that as torpedoes became more sophisticated, more inputs would be required. As the number of spindles increased, the greater complexity meant greater chances of malfunction. Spindle retraction time would be increased even if all systems worked properly. The mechanical transfer of information had to be replaced by electrical connections. This concept became a necessity when the Mark 37 torpedo’s design demanded instantaneous multiple inputs. Finally, there was the need for automatic torpedo firing and torpedo spread setting.
In 1913, Corbin, an authority on submarines at the time, predicted that submarine-to-submarine warfare would remain an impossibility. This opinion was to continue through the Second World War. In part, the opinion was predicated on the limitations of the steam torpedo. Also, sonar equipment prior to the Second World War could not furnish an accurate enough bearing to allow shooting a straight-running steam torpedo with any expectation of a hit. During the war, American submarines had sunk several Japanese submarines, but these attacks were periscope attacks against surfaced submarines. At the end of that war, a British submarine managed to accomplish the feat of hitting and sinking a submerged German submarine.
Arma Corporation tackled submarine officers’ tactical frustrations in a series of TDC improvements ultimately leading to a synthesized system designated the Mark 101 fire control system. It was augmented by the Mark 106 system, which provided for electrically fed inputs to torpedoes. The design of the Mark 101 system was grounded in the several innovative additions that were serially added to the TDC. A review of these TDC components illustrates the progression of bearings-only fire control equipment.
An initial addition to the TDC was a receiver section, which was physically located between the position keeper and angle solver. It received corrected sonar bearing inputs and furnished information to a sonar dial display together with other information such as observed optical bearing, radar bearing, and target range. The receiver section permitted inputs of searchlight sonar bearings to allow integrated best bearing information to the TDC.
The position indicator Mark 6 was the display unit of the receiver section. The main function of the position indicator was to display to conning tower personnel the fire control problem being solved by associated fire control equipment in the attack center of the submarine. The position indicator provided information as it was being received from various sources, the most important of which was sonar. The system also provided for a sound bearing converter that accommodated the difference between the speed of sound through water and the atmosphere.
Another addition was automatic screw noise baseline conversion in which a sonar baseline constant was permanently set into the TDC’s internal mechanism at the time of installation. From these inputs the converter continuously generated sonar baseline corrections, which were angle corrections to accommodate the difference in linear length from the location of a submarine’s screws to the middle of the target.
As the TDC improved system matured into a complete display of own ship movements, target movements and anticipated target turns, Arma engineers developed a console that would totally replace the TDC. The central function of the new Mark 101 display was the Mark 7 analyzer. It was basically an electric-mechanical navigational plotter. It received inputs of own ship’s motion with target ranges and bearings which were displayed together with target course and speed. The accuracy of the solution was dependent upon the accuracy of sonar bearing change rate; the greater the change, the more accurate the solution. The Mark 7 analyzer provided an end-point type of solution, which required a range and a bearing. Two observations were required and a time lapse between bearing change observations of about 90 seconds was required for a reliable solution. The drop-last feature could be utilized to increase the time between observations and could thereby refine the solution. The bearings-only type of solution was used when only target bearings were available. Bearing changes of at least 4.25 degrees were required for a reliable solution. After the initial solution based on three bearings was obtained, and subsequent bearings were entered, the drop-last feature could be utilized to refine the solution. The Mark 7 analyzer was helpful in obtaining quick solutions for target course depending on the reliability of inputs being used. Fire control personnel often used the Mark 7 solution as confirmation of plot-obtained information.
As the Mark 7 analyzer operator entered a series of three target bearings taken at equal intervals as described above, he adjusted estimated target range, course, and speed as further bearings became known. Initial estimates from plots could be integrated into the Mark 7 solution. The system’s analyzer could fit a straight-line constant speed track across the bearings. In effect, the Mark 101 system accomplished an Ekelund speed strip plot within its analog computing ability. The display information was still by dials, but the information was superior to that of the TDC even with multiple improvements.
The Mark 101 console was bulky and best suited to a dedicated space. Some GUPPY III conversions housed the massive console in an engine room which had been converted into an attack center by removal of the engines. The GUPPYs were stretched and pushed in experimental efforts to house the Mark 101/106 and sonar interpretation equipment.
The electric setting of tactical data into tube-loaded torpedoes from Mark 101 fire control solutions was accomplished by the Mark 106 system. The Mark 106 system also provided for tracking of multiple targets. This augmentation provided for more than one submarine in coordinated ASW attacks.10 Of course, the modification of torpedo tubes from spindle settings to electrical settings had to be accompanied by equivalent modifications of the torpedoes themselves. The conversion to electrically set torpedoes meant that complex information could be entered immediately prior to firing.
The finalized system was first installed aboard an operating submarine in 1951. Its central feature was a control console which provided for automatic shifting of spread order from right to left as changes in angle-on-the-bow moved from right to left and vice versa; automatic application of corrections to torpedo tactical data and automatic spread solution and application were inserted into the angle solver. The almost exclusive purpose of these sweeping improvements was to provide fire control capability against submerged submarines.
Since range continued to be a difficult problem even for the Mark 101 fire control system, the submarine force entertained the possibility of placing two hydrophones at the extreme ends of the GUPPY. The concept was simple. With a 300-foot separation, assuming a beam aspect to the target bearing, a triangulation of signal strength might produce a focal point (range) if the target was close enough to allow a signal comparison. To test the theory the Naval Ordnance Laboratory at White Oak, Maryland, used two modified JT hydrophone arrays mounted on the deck of a GUPPY submarine. One was mounted on the bow and the other at the extreme stern. This provided a triangulation of a submerged target at a range short enough to be computed by electronic trigonometry. The system was called “Passive Underwater Fire Control Feasibility Study, or PUFFS. Many GUPPYs of the late 1950s were equipped with PUFFS ranging equipment. Four of the Tang-class boats were also so equipped. These were all deck-mounted hydrophones which produced drag and were prone to environmental interference. The system was improved by moving the hydrophones into tanks, but the final designs on single-hull submarines had side-mounted passive sonar built into the basic structure of the hull.
In the early 1970s, a few Guppy III conversions, such as Tiru (SS-416), included a Mark 66 console for the newly-introduced Mark 48 torpedo. It used the BQS-4 azimuth range indicator. Another part of the system was a Mark 19 plotter for TMA estimates and PUFFS plotting. In these stretched conning tower conversions the PUFFS electronics BQG-4 display was installed. Also in a few attack center conversions were the BQS-4a and BQR-2b displays.
The GUPPY submarine also was America’s first attempt at electronic surveillance of Soviet communications. In the Atlantic, boats from New London and Norfolk put into Portsmouth, England, took on supplies, and headed north through the Irish Sea to the bleak and hazardous waters of the Barents Sea. On board were “spooks” from classified authority who carried black boxes as they crowded the radio space of the control room. Submarine captains received their orders from ComSubLant, who, when defining the special operation mission, tended to be a little vague as to respecting the international 12-mile limit. The Soviet Union claimed a 200-mile limit, which was ludicrous and therefore ignored. The trip was made through state five seas, and those on the bridge of step-sail GUPPYs were strapped to the TBT as the boat plunged through one wave and crested the next. The after room suffered from screws lifting out of water and vibrating their struts. At two-thirds on two engines using the raised snorkel and with the sea coming from off the bow, the submarines plowed their way forward on the surface.
One boat keeping to an eastward course rolled 102 degrees from a beam wave. It was saved by the helmsman, who acted quickly and without orders by putting the rudder hard over, bringing the boat’s bow into the sea. The submarine slowly righted itself, but the lookout and officer of the deck were drowned.
The USS Cochino was lost, and that story was told by Sherry Sontag and Chris Drew in their book, Blind Man’s Bluff. The following abbreviated description of the event has been taken from that book:
It was the need for stealth that convinced intelligence officials that submarines could be the next logical step in the creation of an eavesdropping network that would circle the Soviet Union. On its way to Murmansk the Cochino was rocked by waves as crewmen braced themselves, grabbing chart tables and overhead pipes. Others lunged to catch sliding coffee cups and tools.
“Cochino was running submerged on the snorkel, but the rough sea made the inherently tricky business of snorkeling impossible. The forward engine room reported that water was pouring into the submarine through the snorkel. The commanding officer sent his executive officer to investigate as the engines were shut down from lack of air. About two minutes later, there was a muffled thud and the submarine shuddered. An electrician saw sparks coming from the after battery compartment. He yelled for the compartment to be evacuated. Then, the commanding officer was informed of a fire in the after battery well. The submarine surfaced and was immediately swept by heavy seas. Another submarine, USS Tusk, in company with Cochino, was signaled by flashing light of the after battery fire and resulting casualties. Several crew members including the executive officer had been injured and remained in the after torpedo room of the submarine. All other crewmembers evacuated the after compartments to escape poisonous fumes. They crowded into the forward torpedo room.
The situation went from bad to worse as another crew member went over the side. After approximately two hours it became apparent to the captain that he would have to abandon his submarine. As fires raged below, USS Tusk came alongside. Cochino began to settle by the stern. A makeshift brow [gangplank] was cleated to both boats and Cochino crewmembers scrambled to the Tusk. Captain Benitez was the last person to leave his sinking submarine. The demise of Cochino was America’s introduction to the dangers of the Cold War.
During the 1950s some submarines were built and converted from fleet-type boats or GUPPYs in response to the need for sonar-specific missions. These included the K boats, which were small listening platforms designed only for sonar picket duty. Conversions from fleet snorkels or GUPPYs extended the bow to allow the larger BQR-4 array. These submarines played an important role in monitoring Soviet submarine transits.
GUPPY submarines were incorporated into ASW hunter-killer groups that included a helicopter-carrying aircraft carrier surrounded by a bent-line screen of ASW destroyers. In the van of these units were one or two submarines stationed about 20 miles ahead of the group. In addition, sonobuoy-equipped helicopters hovered on station in a 40-mile semicircle ahead of the main group. These units were very effective ASW threats to any Soviet transiting boats.
In the late 1950s, as an exercise test, a snorkeling GUPPY submarine zigzagged unannounced into an ASW hunter-killer search area. The submarine was detected at an estimated 23,000 yards. It was tracked by another GUPPY using only passive sonar, and the ASW submarine was able to close to a 2,000-yard range. The ASW submarine did not sight the target’s snorkel until just at the firing point. In another 1954 exercise off Iceland, the Cavalla, a converted SSK, simulated a transit to a forward base. The exercise called for the submarine K-1 to be the target and Cavalla the attacker. Both relied entirely on passive sonar. K-1 used the new Target Motion Analysis (TMA) technique and was able to successfully track Cavalla’s movements without Cavalla detecting K-1.
Yard overhauls with equipment and hull modifications kept pace with new sonar and fire control technologies for the duration of the GUPPY era. As described above, a ten-foot extension of the conning tower provided space for the latest TMA equipment, but there were limits to what a fleet-type hull could accommodate. Extension of the conning tower with added weight of equipment meant a higher center of gravity. The safety tank, which had been designed to counter the effect of a flooded conning tower, was rendered useless. The enormous sail produced additional underwater drag and its broad vertical face when on the surface invited heavy rolls in high winds and rough seas.
Some GUPPYs of the 1970s also had a Prairie-Masker system, which pumped bubbles into the screw blades’ leading and trailing edges. This system broke up the cavitation-producing collapsing bubbles, which quieted the boat when running at shallow depths.
The GUPPY program lasted for about twenty-five years, from the late 1940s to the late 1970s. As sufficient nuclear-powered submarines came into the fleet, the number of diesel-powered submarines dwindled. The transition from diesel-electric powered submarines to an all-nuclear powered submarine fleet may have covered over two decades, but the engineering effort to perfect a superior nuclear-powered attack submarine was multidimensional and complex in the extreme. The driving force of the transition was the Cold War evolution of mission definition, which demanded prolonged transiting at high speed and acquisition of submerged targets at great distances.
The Cold War was a race of technology between the United States and the Soviet Union. Each country tried to embarrass the other by attempting to force the opposite country’s submarines to the surface, thus exposing espionage efforts. It was commonly known, for example, that ComSubLant offered a case of Jack Daniels to the submarine that could force a Soviet Foxtrot boat to the surface. But, although the enthusiasm on both sides was extreme, the contest between the United States and the Soviet Union for world dominance never reached a level of open conflict.
As a result, sophisticated submarine torpedo fire control techniques, computers with high-speed TMA solutions, and the ability to diagnose noise signatures coming from opponents’ submarines never produced a single warshot torpedo fired from one submarine at another. It is remarkable that in 25 years of brinksmanship so intense that submarines were sunk, none did so from a torpedo attack. The casualties of the submarine Cold War resulted only from accidents in training exercises, equipment failures and aggressive surveillance collisions.
In a review of submarine torpedo fire control during the Cold War, it is appropriate to examine submarine development as a platform for torpedoes. Parenthetically, the decommissioning of the last diesel-electric powered submarine in the Navy’s fleet was later to bring about an unusual arrangement with a foreign nation.