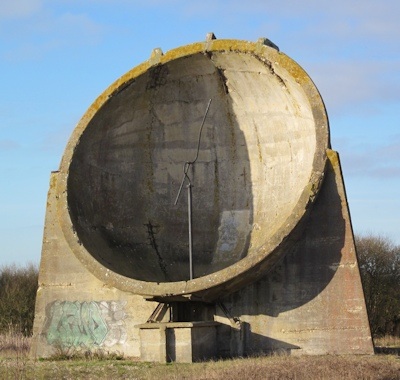
30ft Sound Mirror
Sir Robert Watson-Watt
Possibly the first group positively to start research into
radio detection was that under Dr A. Hoyt Taylor at the US Navy Radio Division
at Anacostia (predecessor of the Naval Research Laboratory, founded in 1923).
In September 1922, probably triggered by Marconi’s recent speech, Taylor and
Leo C. Young did experiments with 5 m (200 in) wavelength ‘radar’ and obtained
startling results from a small wooden steamship. They wrote the Navy Bureau of
Engineering an historic memo which, among other things, mentioned detection of
aircraft. The report was filed and forgotten. But Taylor and Young kept picking
away at the problem and in 1930, in the course of radio DF experiments, noticed
reflections from aircraft and measured the range. The result was a second memo,
Radio Echo Signals from Moving Objects, dated November 1930. This time it was
not ignored. The US Army was brought in, and Colonel William R. Blair, who had
for years been prodding a team trying to replace the sound locator by either a
heat detector or radio waves, attacked the problem of aircraft detection with
renewed vigour on the basis of the Navy report. His Signal Corps laboratories
at Fort Monmouth, New Jersey, began working round the clock trying to build a
radar not only using metre waves but also the much shorter (centimetric) waves
now known as microwaves. These experiments, the first in the world on microwave
radar, produced clear echoes from nearby targets (see diagram below). However,
at that time there was no known way of generating microwaves except at
extremely small powers, quite useless for practical purposes. Such waves could
be generated by various means, including Hull’s magnetron, but it took until
well into the Second World War before British workers achieved the vital
breakthrough and generated high-power microwaves.
In 1933, while a team that could be counted on the fingers
was toiling at Fort Monmouth, another team got into the act in the
communications research department of the German Navy. This had nothing to do
with the coming to power of the Nazis under Hitler, and the opening of the
financial floodgates for new German armaments. It was simply the gradual
belief, fostered in the mind of Dr Rudolph Kühnold, department head, that if
sonar worked with acoustic waves under water, radio waves ought to do the same
in the atmosphere. (Exactly the same idea occurred to King George V; after
listening to an Admiralty lecture on Asdic and echo-sounding, he asked the
speaker if the same thing could be done with radio waves against aircraft, receiving
the reply that, so far as was known in 1931, it could not). Kühnold, knowing
little of the wealth of prior work in the field, boldly plunged into the
challenging field of microwaves. Late in 1933 he obtained a marvellous new
magnetron valve from the Dutch Philips works at Eindhoven. It could generate 70
watts at 600 MHz, equivalent to a wavelength of about 0.5 metre, thousands of
times more powerful than had previously been possible. A good radar was quickly
made as a ‘breadboard’ (an untidy, non-portable laboratory lash-up), and in
January 1934 the GEMA company was formed to accelerate this now highly secret
work. By March 1934 a complete set was operating in a room overlooking Kiel
harbour, with dish aerials on the balcony aimed at the old battleship Hessen.
With a few changes to prevent the receiver from being flooded with radiation
from the adjacent transmitter, the GEMA team got an excellent picture.
Work now went ahead fast, the GEMA team was moved to a
bigger research establishment at Pelzerhaken, near Lübeck, and soon pulsed
radars were giving good results against both ships and aircraft. By 1936 the
GEMA team, now expanded to many hundreds, had the prototypes of two of the most
important German radars of the Second World War. One was the big Seetakt
gunnery radar for major surface vessels. So poor was British intelligence in
1938 that it did not notice the huge bedstead aerial of Seetakt on the three
‘pocket battleships’ and then on the battle-cruisers Scharnhorst and Gneisenau.
The Royal Navy fondly believed itself the only navy to have heard of radar,
even though it was not due to get the first British equivalent of Seetakt until
1941. The other GEMA set was even more important: it was a mobile land-based
early-warning set for use against aircraft, with 360° cover and range up to an
unprecedented 120 km (75 miles). Named Freya, after the Norse goddess, it went
into large-scale production for the Luftwaffe and German Navy and was by far
the most important German early-warning radar until the end of 1942.
Nor was this all. By 1936 the concept of radar had moved
right out of the laboratory in Germany and become a weapon that could be
specified and put into production. The Luftwaffe’s rapidly growing Flak (AA
artillery) wanted an extremely accurate radar for blind gunfire at night or
through cloud, to complement the superb guns it was receiving of 88, 105 and
128 mm calibre. The answer was a first-class set developed by Lorenz and
Telefunken, the Würzburg, operating at about 0.5 metre on 560 or 570 MHz. Nowhere
else in the world in 1937 was there a radar giving such good discrimination and
precision, because nowhere else could one be made to operate with such power (8
kW) at such a high frequency and short wavelength. Indeed, special versions
tested in 1938 generated a power of 50 kW. These were the best general-purpose
mass-produced radars in the world before the Second World War. British
intelligence never noticed the rotating dish aerials, nor did it detect the
transmissions or get into helpful talk with the crews. In November 1939 a
well-wisher gave a fat parcel to the British naval attaché in Oslo. Among
masses of highly secret information it contained on German developments were
details of the Freya, Würzburg and other radars, and of guidance beams used by the
Luftwaffe to aid night bombers. The British authorities dismissed the report as
a trick. True, the facts already known to them tallied; but that was to make
Britain fall for the rest. Everyone knew the Germans had no radar!
This unbelievably smug attitude did not extend to the
outstanding team working on radar in Britain. This team had many beginnings in
many places, but it was drawn together in a sensible way by the not untimely
belief, in 1934, that perhaps all was not well with Britain’s air defences.
There was no way of detecting hostile bombers at a distance; defending fighters
had to fly exhausting ‘standing patrols’ where it was thought that the enemy
might appear. The sole attempt to find a new method had been the construction
on Romney Marshes, on the southern shore of Kent, of a 220-foot concrete ‘sound
intensifier’ intended to pick up the sound of distant bombers (there was
another, not far away, which was never finished). The Romney Mirror could just
about do its job if the bombers were expected, and there was no background
noise of birds or motor-boats. It could not, of course, say anything about the
raiding force’s height or range, numbers or direction; and the effective range
varied from short to useless. The bombers also had to cooperate by approaching
from the right direction. As in the early 1930s the only large bomber force on
the local part of the Continent was French, the structure’s axis was directed
towards Paris. As the eventual ‘father of radar’ Sir Robert Watson-Watt put it,
‘Reinforced concrete is rudely uncompliant to the winds of diplomacy.’
Nobody was really satisfied with the giant Romney Mirror,
even in the days of slow bombers. One who appreciated the gravity of the
situation was Harry Wimperis, the first Director of Scientific Research (DSR)
at the Air Ministry in London. In June 1934 his Personal Scientific Assistant,
A.P. Rowe, sought solace in researching the whole subject of science as applied
to air defence. He was merely interested; he had not been asked to do this. He
ultimately unearthed fifty-three files on the matter. Lest this should sound
encouraging, there were at that time several times 53 thousand files in the Air
Ministry on other topics; and many of the fifty-three contained mere brief
letters or one-page memoranda. Rowe was deeply concerned. ‘Clearly,’ he
concluded, ‘little or no effort had been made to call on science to find a way
out. I therefore wrote a memorandum summarizing the unhappy position, and
proposing that the DSR should tell the Secretary of State for Air of the
dangers ahead. The memorandum said that unless science evolved some new method
of air defence, we were likely to lose the next war if it started within ten
years. Unfortunately, I was not clever enough to think of a new method.’
When one reflects on the situation the mind boggles at how
it could have come about. The need for a method of unfailingly detecting,
locating, and tracking enemy aircraft had been clear and obvious from 1915
onwards. There surely appeared to be only a very limited range of possible
methods, each derived from heat, light, sound, magnetism or electricity
(accepting further that heat, light, magnetism and electricity are all
manifestations of one of the most fundamental phenomena in the universe, the
electromagnetic wave). Any bright schoolboy asked to think about the matter
would have gone to see eminent physicists, radio and acoustics engineers, and
the matter would have snowballed as each worker named others prominent in the
same field. Within twenty-four hours our schoolboy would have heard of Hertz,
and of the shoal of subsequent workers who had demonstrated the reflective
properties of radio waves and patented Telemobiloscopes. He would hardly have
been able to avoid discovering that as lately as 1932 four engineers
researching VHF (very high frequency) radio for the Post Office had included in
their large published report how cross they had been at interference from
aircraft, describing the measured aircraft ranges and the way the
beat-interference varied with the aircraft’s speed. In 1933 Bell Telephone
Laboratories in the United States published even more extensive observations.
Yet here was Rowe, in June 1934, armed with the sum total of knowledge of the
British Air Ministry still unaware of any of these things, yet feeling there
must be a better way than a 220-foot ‘sound mirror’ made of concrete.
Wimperis read his report and in November 1934, after an
unexplained wait of half a year, suggested to the Secretary of State that there
should be a Committee for the Scientific Survey of Air Defence. The CSSAD was
duly formed, but before its first meeting Wimperis telephoned a man who knew a
lot about radio waves, Robert Watson-Watt, Superintendent of the Radio
Department at the National Physical Laboratory at Teddington. Virtually the
only new kind of air defence anybody could think of in 1934 was the ‘death
ray’. Since the First World War inventors had come to the British government at
frequent intervals with paper proposals and even with secret black boxes
promising wondrous things. Eventually the Air Ministry offered £1,000 cash (a
fortune then) to anyone who could kill a sheep with such a box at 100 yards (91
m), the secret to remain with the owner. No sheep was so much as singed. But,
not knowing what else to do, Wimperis asked Watson-Watt whether such a ray was
possible. Again one is amazed that the Air Ministry had never asked before.
‘Wattie’ could merely have answered ‘no’. But, having
devoted a minute’s thought to the problem, he could see that the first thing
one had to do with a death ray was to ascertain the exact direction of the
hostile aircraft. As the radio energy sent out would otherwise have to be
millions to billions of times the energy hitting the target, knowing the
latter’s position was all-important. So the radio expert – while pointing out
that in January 1935 there was no known way of generating sufficient radio
energy at sufficiently short wavelength even ‘to make the pilot’s blood boil’,
let alone destroy a bomber – added that he had also considered the ‘still
difficult but less-unpromising problem of radio-detection as opposed to
radio-destruction, and numerical considerations on the method of detection by
reflected radio waves will be submitted if desired’. This unsolicited offer was
the start of what is probably the biggest and most widespread revolution in the
history of warfare.
Things moved fast. On 10 February Wimperis asked for the
‘numerical considerations’, and Watson-Watt and his colleague Arnold Wilkins
worked them all out and submitted them in an historic memorandum dated 12
February 1935. The answer came out unexpectedly favourable – so much so that
W-W wrote ‘I am still nervous as to whether we have not got a power of ten
wrong, but even that would not be fatal.’
In this document W-W described much of radar as it has
existed up to the present day. He showed how the airframe of an aircraft (not
just a metal-skinned one) would behave when flooded with short-wave radio
energy as a conducting receiver aerial, and thus also as a transmitter. The
observers on the ground would scan the skies with short-wave energy until they
found a target; then, using techniques that the Radio Department had already
devised, they would track it automatically and measure its range, bearing and
height. W-W himself had many years previously invented an instantaneous-reading
cathode-ray direction finder (CRDF), showing on a CRT the direction of a
distant thunderstorm. There seemed to be no barrier to refining the technique
to give immediate, and more accurate, ‘radio location’ of an aircraft, with two
CRTs showing range, bearing and elevation (and thus height). Use of radio
pulses would allow the transmitter valves to pump out enormously greater bursts
of power, and could also assist range measurement. On the other hand, there was
a technique of using continuous-wave (CW) radiation that would enable moving
objects alone to be detected (so-called moving-target indication, MTI, which
was eventually put to use long after the Second World War). By no means least,
there was a need for an identification friend-or-foe (IFF) method, so that
defenders would know when to shoot; this too, wrote Watson-Watt, could be
provided.
In this document – as important to British history as Magna
Carta – he described not just the basic underlying principles of radar, which
had never before been set forth so comprehensively, but also the way a complete
British defence system should be constructed, approximately how it would
perform, and how the complete system – with communications to plotting centres
and to fighter pilots, IFF and even enemy anti-radar countermeasures – would
eventually function. Not the least thing about it was its air of total
authority. Watson-Watt, in precise and official language, was describing techniques
and often even existing hardware that he and his colleagues had devised, built
and used. This was no ‘mad inventor’ with a harebrained and unexplainable
secret, but a mature engineer with a proposal that was wholly valid, wholly
explainable and capable of swift verification. By no means least, it was
enormously encouraging; Watson-Watt promised, ‘The amount of reflected energy
you will get back is amazingly big.’ How the whole thing seemed to an Air Staff
previously barren and desolate of ideas can be imagined.
Within days Watson-Watt had seen the Air Member for Research
and Development, Air Marshal Hugh (later Sir Hugh) Dowding. On 26 February a
Handley Page Heyford bomber, with light-alloy structure covered by fabric,
shuttled up and down a twenty-mile path, passing each time approximately over a
very ordinary field in Northamptonshire. Unknown to the pilot, the Heyford was
in the centre of the powerful short-wave Empire radio beam from the BBC radio
station at Daventry. In the field was a huddle of figures. Watson-Watt, Wilkins
and A.P. Rowe quietly watched a tiny stub of green light on a CRT grow longer
and then shorter, as the bomber reflected back the radiation. With crude and
hastily thrown-together equipment they were detecting the Heyford at a range of
up to eight miles. As Watson-Watt drove Rowe back to London they discussed a
future that seemed open-ended – if their country was granted enough time. From
the fairly short-term possibility of early-warning over ranges of at least
sixty miles, and possibly 200, could be derived short-range precision guidance
for searchlights and guns, and possibly, one day, a radar that could be carried
aloft in a fighter. But Watson-Watt, a frequent visitor to a Germany now ruled
by the Nazis, did not think he could be sure of having more than two years to
turn talk into reality.
It is commonly thought that British government circles,
especially in 1935, either tended to take negative decisions or to put off
taking any at all. This was emphatically not the case with radar. Though the
first RAF exercise using the new invention, in September 1936, tended to throw
up problems rather than successes, Britain was by this time officially ‘sold’
on a completely new defence system, had voted money for the start of an operational
chain of five stations and was already building them. Basic construction was,
in fact, the one thing that held the programme back and prevented good results.
On the technical side there was a mixture of sheer brilliance and sheer luck.
One of the lucky things was Watson-Watt himself: he was possessed of fantastic
energy, drive and infectious enthusiasm that until 1945 sustained a pace of
research that continually astonished his political masters. Another was that he
did not think of his future hostile aircraft as small spheres (as many experts
did) but as horizontal pieces of wire. His original calculation determined the
current that would be induced in this wire from wing tip to wing tip, which not
only gave an encouraging result but set the team working on a wavelength of 50
metres, twice a typical bomber wing span. Had he regarded aeroplanes as spheres
he would have chosen a much shorter wavelength, and no good results would have
been attained for years – by which time the Air Staff and government would have
gone back to the fruitless chase after sound or heat detection, losing the
Second World War before the end of 1940.
Another mix of luck and brilliance concerned the design of
the hardware. There were an almost infinite number of ways of arranging a radar
station, and the team that was eventually set up as Air Ministry Research
Establishment (AMRE) at Bawdsey Research Station, on the remote Suffolk coast,
hardly put a foot wrong. Sticking at first to the long 50 m wavelength, they
designed each station to be monostatic, serving as a self-contained transmitter
and receiver. Watson-Watt hit on a brilliant method of using multiple crossed
dipole aerials so that each station could determine the exact direction of each
target, with a PPI (plan-position indicator) giving its immediate bearing. The
team’s already existing CRDF was a godsend in giving the necessary background
of experience. Each station did not send out a directional beam, which would
have had to rotate like a lighthouse, but behaved like a floodlight, pumping
out pulses in all directions; eventually 90 per cent went outwards, out over
the sea. Special circuits indicated whether a target was in front or behind.
With some difficulty, bothered at first by the Earth’s surface and some unexpected
reflections from the lower parts of the ionosphere, it became possible very
accurately to measure elevation angle and thus target height. By mid-1936 all
the elements could be said to work, when properly set up and calibrated by
experts – though not when used by anyone else, a feature that was long
characteristic of this new, secret and often amazing invention, the first and
greatest creation of the select race soon to be called ‘boffins’.
At first all the emphasis went into the emergency building
of this single chain, like a modern castle wall round the British south and
east coast. Its original name was RDF (radio direction finding, a plausible
cover), later changed to radiolocation (which revealed what it did) and in
January 1944 standardized with the United States as the name by which the world
knows it today, radar. This initial chain used 240- and 320-foot towers serving
fantastically powerful transmitters, made mainly by Metropolitan-Vickers and
containing huge valves devised by HM Signals School at Portsmouth. To the
latter’s horror, Bawdsey ran the filaments far too hot, pumped in more than
double the voltage, and instead of the rated power of 10 kW pushed the output
by stages to almost 200 kW! Combined with the equally rapid progress with the
receiver, made chiefly by Cossor, the 1936 RDF station could detect aircraft at
well over one hundred miles, at any attainable altitude, and indicate whether
the ‘blip’ (the small spike of light denoting the target) was a single
aircraft, two, three, or more than three. (By 1940 girls of the WAAF had become
so expert they could even give a reliable approximation of the strength of a
large formation.) The only obvious shortcoming was that the system could be
jammed. In early 1938 Bawdsey’s E.C. Williams flew offshore in the prototype
Sunderland flying boat, then on trials at Felixstowe, sending out powerful
waves of interference from a modified hospital diathermy apparatus. Within days
his colleagues were making the first operational radar in the world resistant to
jamming – the start of the mighty science of ECM, electronic countermeasures.
By this time the 50 m waves had been halved in length, and four separate
frequencies could be chosen by each station to defeat hostile ECM.
While this ‘RDF 1’ went from strength to strength,
Watson-Watt – many said Sir Henry Tizard thought of it – lost little time in
beginning ‘RDF 2’. His reasoning, immediately backed up by Tizard, was so
obvious it might have been overlooked. He had no doubt RDF 1 would do its job.
By giving early warning and location of every enemy raid it would, when
combined with a proper reporting and control organization, eliminate the need
for standing patrols and enable every available fighter to make a sure
interception – something that had never before been possible. It would enable
the day raider to be defeated, which was just what the Air Staff had been
trying to do for twenty years. So what would happen next? Why, the enemy would
come only in foul weather or by night. Day fighters could not even fly safely
under such conditions. Even if they could, they could no longer make a sure
interception and get the enemy in their sights. So RDF 2 was a project to put a
radar set in a fighter. Compared with RDF 1 it was several times more
difficult.
RDF 1 sent out giant pulses from an aerial system about as
big as a football field, supported by huge towers. To provide the energy, a
transmitter about as big as a house took as much current as a small town, and
the reflected pulses were picked up by an equally large receiver manned by a
team of experts. All this was a bit much to put inside a fighter. It obviously
meant starting again on a shorter wavelength and, somehow, slashing the weight
to turn tons into pounds. It was indeed a formidable undertaking.