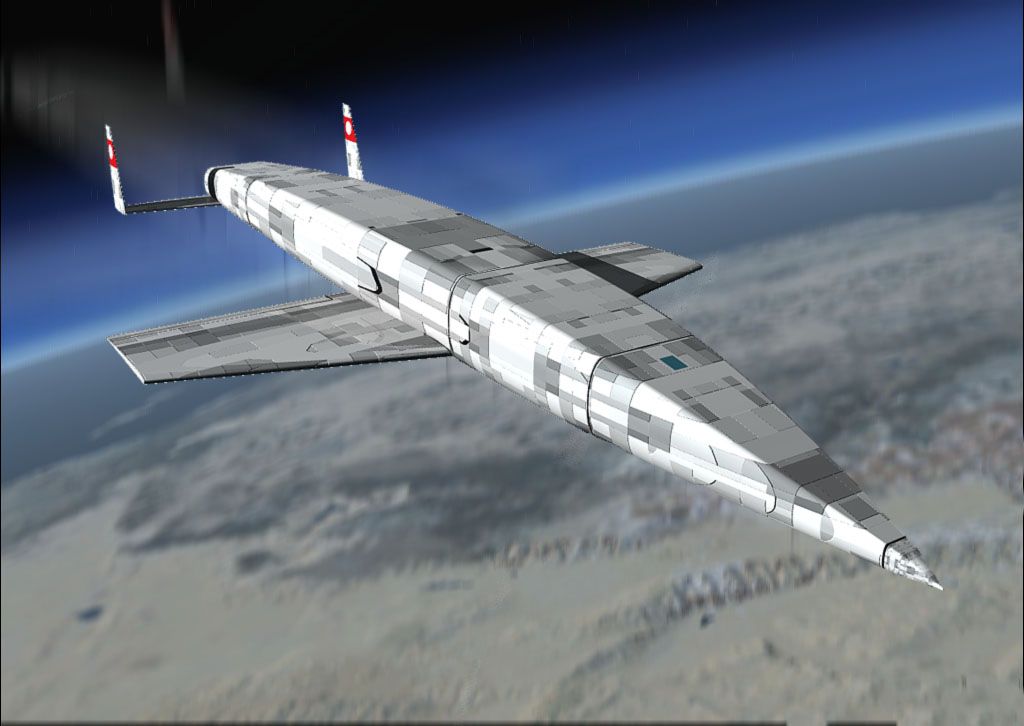
Sanger Amerika Bomber
Today’s world of high-speed flight is international, with
important contributions having recently been made in Japan, Australia, and
Russia as well as in the United States. This was even truer during World War
II, when Adolf Hitler sponsored development programs that included early jet
fighters and the V-2 missile. America had its own research center at NACA’s
Langley Memorial Aeronautical Laboratory, but in important respects America was
little more than an apt pupil of the wartime Germans. After the Nazis
surrendered, the U.S. Army brought Wernher von Braun and his rocket team to
this country, and other leading researchers found themselves welcome as well.
Some of their best work had supported the V-2, using a pair
of tunnels that operated at Mach 4.4. This was just short of hypersonic, but
these facilities made a key contribution by introducing equipment and research
methods that soon found use in studying true hypersonic flows. At Peenemunde,
one set of experiments introduced a wind-tunnel nozzle of specialized design
and reached Mach 8.8, becoming the first to achieve such a speed. Other German
work included the design of a 76,000-horsepower installation that might have
reached Mach 10.
The technical literature also contained an introductory
discussion of a possible application. It appeared within a wartime report by
Austria’s Eugen Sänger, who had proposed to build a hypersonic bomber that
would extend its range by repeatedly skipping off the top of the atmosphere
like a stone skipping over water. This concept did not enter the mainstream of
postwar weapons development, which gave pride of place to the long-range
ballistic missile. Still, Sänger’s report introduced skipping entry as a new
mode of high-speed flight, and gave a novel suggestion as to how wings could
increase the range of a rocket-powered vehicle.
Within Langley, ongoing research treated flows that were
merely supersonic. However, the scientist John Becker wanted to go further and
conduct studies of hypersonic flows. He already had spent several years at
Langley, thereby learning his trade as an aerodynamicist. At the same time he
still was relatively young, which meant that much of his career lay ahead of
him. In 1947 he achieved a major advance in hypersonics by building its first
important research instrument, an 11-inch wind tunnel that operated at Mach
6.9.
German Work with
High-Speed Flows
At the Technische Hochschule in Hannover, early in the
twentieth century, the physicist Ludwig Prandtl founded the science of
aerodynamics. Extending earlier work by Italy’s Tullio Levi-Civita, he
introduced the concept of the boundary layer. He described it as a thin layer
of air, adjacent to a wing or other surface, that clings to this surface and
does not follow the free-stream flow. Drag, aerodynamic friction, and heat
transfer all arise within this layer. Because the boundary layer is thin, the
equations of fluid flow simplified considerably, and important aerodynamic
complexities became mathematically tractable.
As early as 1907, at a time when the Wright Brothers had not
yet flown in public, Prandtl launched the study of supersonic flows by
publishing investigations of a steam jet at Mach 1.5. He now was at Göttingen
University, where he built a small supersonic wind tunnel. In 1911 the German
government founded the Kaiser-Wilhelm-Gesellschaft, an umbrella organization
that went on to sponsor a broad range of institutes in many areas of science
and engineering. Prandtl proposed to set up a center at Göttingen for research
in aerodynamics and hydrodynamics, but World War I intervened, and it was not
until 1925 that this laboratory took shape.
After that, though, work in supersonics went forward with
new emphasis. Jakob Ackeret, a colleague of Prandtl, took the lead in building
supersonic wind tunnels. He was Swiss, and he built one at the famous
Eidgenossische Technische Hochschule in Zurich. This attracted attention in
nearby Italy, where the dictator Benito Mussolini was giving strong support to
aviation. Ackeret became a consultant to the Italian Air Force and built a
second wind tunnel in Guidonia, near Rome. It reached speeds approaching 2,500
miles per hour (mph), which far exceeded those that were available anywhere
else in the world.
These facilities were of the continuous-flow type. Like
their subsonic counterparts, they ran at substantial power levels and could
operate all day. At the Technische Hochschule in Aachen, the aerodynamicist
Carl Wiesenberger took a different approach in 1934 by building an
intermittent-flow facility that needed much less power. This “blowdown”
installation relied on an evacuated sphere, which sucked outside air through a
nozzle at speeds that reached Mach 3.3.
This wind tunnel was small, having a test-section diameter
of only four inches. But it set the pace for the mainstream of Germany’s
wartime supersonic research. Wieselberger’s assistant, Rudolf Hermann, went to
Peenemunde, the center of that country’s rocket development, where in 1937 he
became head of its new Aerodynamics Institute. There he built a pair of large
supersonic tunnels, with 16-inch test sections, that followed Aachen’s blowdown
principle. They reached Mach 4.4, but not immediately. A wind tunnel’s
performance depends on its nozzle, and it took time to develop proper designs.
Early in 1941 the highest working speed was Mach 2.5; a nozzle for Mach 3.1 was
still in development. The Mach 4.4 nozzles were not ready until 1942 or 1943.
The Germans never developed a true capability in
hypersonics, but they came close. The Mach 4.4 tunnels introduced equipment and
methods of investigation that carried over to this higher-speed regime. The
Peenemunde vacuum sphere was constructed of riveted steel and had a diameter of
40 feet. Its capacity of a thousand cubic meters gave run times of 20 seconds.4
Humidity was a problem; at Aachen, Hermann had learned that moisture in the air
could condense when the air cooled as it expanded through a supersonic nozzle,
producing unwanted shock waves that altered the anticipated Mach number while
introducing nonuniformities in the direction and velocity of flow. At
Peenemunde he installed an air dryer that used silica gel to absorb the
moisture in the air that was about to enter his supersonic tunnels.
Configuration development was at the top of his agenda. To
the modern mind the V-2 resembles a classic spaceship, complete with fins. It
is more appropriate to say that spaceship designs resemble the V-2, for that
missile was very much in the forefront during the postwar years, when science
fiction was in its heyday. The V-2 needed fins to compensate for the limited
effectiveness of its guidance, and their design was trickier than it looked.
They could not be too wide, or the V-2 would be unable to pass through railroad
tunnels. Nor could they extend too far below the body of the missile, or the
rocket exhaust, expanding at high altitude, would burn them off.
The historian Michael Neufeld notes that during the 1930s,
“no one knew how to design fins for supersonic flight.” The A-3, a test missile
that preceded the V-2, had proven to be too stable; it tended merely to rise
vertically, and its guidance system lacked the authority to make it tilt. Its
fins had been studied in the Aachen supersonic tunnel, but this problem showed
up only in flight test, and for a time it was unclear how to go further.
Hermann Kurzweg, Rudolf Hermann’s assistant, investigated low-speed stability
building a model and throwing it off the roof of his home. When that proved
unsatisfactory, he mounted it on a wire, attached it to his car, and drove down
an autobahn at 60 mph.
The V-2 was to fly at Mach 5, but for a time there was
concern that it might not top Mach 1. The sound barrier loomed as potentially a
real barrier, difficult to pierce, and at that time people did not know how to
build a transonic wind tunnel that would give reliable results. Investigators
studied this problem by building heavy iron models of this missile and dropping
them from a Heinkel He-111 bomber. Observers watched from the ground; in one
experiment, Von Braun himself piloted a plane and dove after the model to
observe it from the air. The design indeed proved to be marginally unstable in
the transonic region, but the V-2 had the thrust to power past Mach 1 with
ease.
A second test missile, the A-5, also contributed to work on
fin design. It supported development of the guidance system, but it too needed
fins, and it served as a testbed for further flight studies. Additional flight
tests used models with length of five feet that were powered with rocket
engines that flew with hydrogen peroxide as the propellant.
These tests showed that an initial fin design given by
Kurzweg had the best subsonic stability characteristics. Subsequently,
extensive wind-tunnel work both at Peenemunde and at a Zeppelin facility in
Stuttgart covered the V-2’s complete Mach range and refined the design. In this
fashion, the V-2’s fins were designed with only minimal support from Peenemunde’s
big supersonic wind tunnels. But these tunnels came into their own later in the
war, when investigators began to consider how to stretch this missile’s range
by adding wings and thereby turning it into a supersonic glider.
Once the Germans came up with a good configuration for the
V-2, they stuck with it. They proposed to use it anew in a two-stage missile
that again sported fins that look excessively large to the modern eye, and that
was to cross the Atlantic to strike New York. But there was no avoiding the
need for a new round of wind-tunnel tests in studying the second stage of this
intercontinental missile, the A-9, which was to fly with swept wings. As early
as 1935 Adolf Busemann, another colleague of Prandtl, had proposed the use of such
wings in supersonic flight. Walter Dornberger, director of V-2 development,
describes witnessing a wind-tunnel test of a model’s stability.
The model had “two knifelike, very thin, swept-back wings.”
Mounted at its center of gravity, it “rotated at the slightest touch.” When the
test began, a technician opened a valve to start the airflow. In Dornberger’s
words,
“The model moved abruptly, turning its nose into the
oncoming airstream. After a few quickly damping oscillations of slight
amplitude, it lay quiet and stable in the air that hissed past it at 4.4 times
the speed of sound. At the nose, and at the edges of the wing supports and
guide mechanism, the shock waves could be clearly seen as they traveled
diagonally backward at a sharp angle.
As the speed of the airflow fell off and the test ended, the
model was no longer lying in a stable position. It made a few turns around its
center of gravity, and then it came to a standstill with the nose pointing
downward. The experiment Dr. Hermann had wished to show me had succeeded
perfectly. This projectile, shaped like an airplane, had remained absolutely
stable at a supersonic speed range of almost 3,500 mph.”
Work on the A-9 languished for much of the war, for the V-2
offered problems aplenty and had far higher priority. But in 1944, as the
Allies pushed the Germans out of France and the Russians closed in from the
east, Dornberger and Von Braun faced insistent demands that they pull a rabbit
from a hat and increase the V-2’s range. The rabbit was the A-9, with its wings
promising a range of 465 miles, some three times that of the standard V-2.
Peenemunde’s Ludwig Roth proceeded to build two prototypes.
The V-2 was known to its builders as the A-4, and Roth’s A-9 now became the
A-4b, a designation that allowed it to share in the high priority of that
mainstream program. The A-4b took shape as a V-2 with swept wings and with a
standard set of fins that included slightly enlarged air vanes for better
control. Certainly the A-4b needed all the help it could get, for the addition
of wings had made it highly sensitive to winds.
The first A-4b launch took place late in December 1944. It
went out of control and crashed as the guidance system failed to cope with its
demands. Roth’s rocketeers tried again a month later, and General Dornberger
describes how this flight went much better:
“The rocket, climbing vertically, reached a peak altitude of
nearly 50 miles at a maximum speed of 2,700 mph. [It] broke the sound barrier
without trouble. It flew with stability and steered automatically at both
subsonic and supersonic speeds. On the descending part of the trajectory, soon
after the rocket leveled out at the upper limit of the atmosphere and began to
glide, a wing broke. This structural failure resulted from excessive
aerodynamic loads.”
This shot indeed achieved its research goals, for it was to
demonstrate successful launch and acceleration through the sound barrier,
overcoming drag from the wings, and it did these things. Gliding flight was not
on the agenda, for while wind-tunnel tests could demonstrate stability in a
supersonic glide, they could not guard against atmosphere entry in an improper
attitude, with the A-4b tumbling out of control.
Yet while the Germans still had lessons to learn about loads
on a supersonic aircraft in flight, they certainly had shown that they knew
their high-speed aerodynamics. One places their achievement in perspective by
recalling that all through the 1950s a far wealthier and more technically
capable United States pursued a vigorous program in rocket-powered aviation
without coming close to the A-4b’s performance. The best American flight, of an
X-2 in 1956, approached 2,100 mph—and essentially duplicated the German failure
as it went out of control, killing the pilot and crashing. No American rocket
plane topped the 2,700 mph of the A-4b until the X-15 in 1961.
Hence, without operating in the hypersonic regime, the
Peenemunde wind tunnels laid important groundwork as they complemented such
alternative research techniques as dropping models from a bomber and flying
scale models under rocket power. Moreover, the Peenemunde aerodynamicist
Siegfried Erdmann used his center’s facilities to conduct the world’s first
experiments with a hypersonic flow.
In standard operation, at speeds up to Mach 4.4, the
Peenemunde tunnels had been fed with air from the outside world, at atmospheric
pressure. Erdmann knew that a hypersonic flow needed more, so he arranged to
feed his tunnel with compressed air. He also fabricated a specialized nozzle
and aimed at Mach 8.8, twice the standard value. His colleague Peter Wegener
describes what happened:
“Everything was set for the first-ever hypersonic flow
experiment. The highest possible pressure ratio across the test section was
achieved by evacuating the sphere to the limit the remaining pump could
achieve. The supply of the nozzle—in contrast to that at lower Mach numbers—was
now provided by air at a pressure of about 90 atmospheres…. The experiment was
initiated by opening the fast-acting valve. The flow of brief duration looked
perfect as viewed via the optical system. Beautiful photographs of the flow
about wedge-shaped models, cylinders, spheres, and other simple shapes were
taken, photographs that looked just as one would expect from gas dynamics
theory.”
These tests addressed the most fundamental of issues: How,
concretely, does one operate a hypersonic wind tunnel? Supersonic tunnels had
been bedeviled by condensation of water vapor, which had necessitated the use
of silica gel to dry the air. A hypersonic facility demanded far greater
expansion of the flow, with consequent temperatures that were lower still.
Indeed, such flow speeds brought the prospect of condensation of the air
itself.
Conventional handbooks give the liquefaction temperatures of
nitrogen and oxygen, the main constituents of air, respectively as 77 K and 90
K. These refer to conditions at atmospheric pressure; at the greatly rarefied
pressures of flow in a hypersonic wind tunnel, the pertinent temperatures are
far lower. In addition, Erdmann hoped that his air would “supersaturate,”
maintaining its gaseous state because of the rapidity of the expansion and
hence of the cooling.
This did not happen. In Wegener’s words, “Looking at the
flow through the glass walls, one could see a dense fog. We know now that under
the conditions of this particular experiment, the air had indeed partly
condensed. The fog was made up of air droplets or solid air particles forming a
cloud, much like the water clouds we see in the sky.” To prevent such
condensation, it proved necessary not only to feed a hypersonic wind tunnel
with compressed air, but to heat this air strongly.
One thus is entitled to wonder whether the Germans would
have obtained useful results from their most ambitious wind-tunnel project, a
continuous-flow system that was designed to achieve Mach 7, with a possible
extension to Mach 10. Its power ratings pointed to the advantage of blowdown
facilities, such as those of Peenemunde. The Mach 4.4 Peenemunde installations
used a common vacuum sphere, evacuation of which relied on pumps with a total
power of 1,100 horsepower. Similar power levels were required to dry the silica
gel by heating it, after it became moist. But the big hypersonic facility was
to have a one-meter test section and demanded 76,000 horsepower, or 57
megawatts.
Such power requirements went beyond what could be provided
in straightforward fashion, and plans for this wind tunnel called for it to use
Germany’s largest hydroelectric plant. Near Kochel in Bavaria, two lakes—the
Kochelsee and Walchensee—are separated in elevation by 660 feet. They stand
close together, providing an ideal site for generating hydropower, and a hydro
plant at that location had gone into operation in 1925, generating 120
megawatts. Since the new wind tunnel would use half of this power entirely by
itself, the power plant was to be enlarged, with additional water being
provided to the upper lake by a tunnel through the mountains to connect to
another lake.
In formulating these plans, as with the A-4b, Germany’s
reach exceeded its grasp. Moreover, while the big hypersonic facility was to
have generous provision for drying its air, there was nothing to prevent the
air from condensing, which would have thrown the data wildly off.20 Still, even
though they might have had to learn their lessons in the hard school of
experience, Germany was well on its way toward developing a true capability in
hypersonics by the end of World War II. And among the more intriguing concepts
that might have drawn on this capability was one by the Austrian rocket
specialist Eugen Sänger.